Geiger-Mueller (GM) Tubes
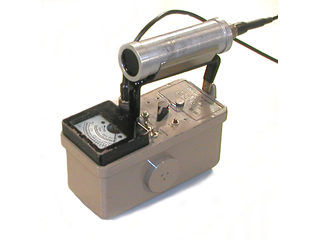
The essential components of the Geiger-Mueller detector are its two collecting electrodes: the anode and cathode (the anode is positively charged with respect to the cathode). In most cases, the outer chamber wall serves as the cathode. The potential difference between the anode and cathode is usually in the 800 to 1200 volt range.
There are three basic GM detector designs:
- Side Window (cylindrical)
- End Window
- Pancake
Side Window (thin/thick walled cylindrical) GM
A cylinder (often 446 stainless steel) serves as the cathode while a wire (usually tungsten) running along the central axis serves as the anode. The primary application of the side window GM is the measurement of gamma exposure rates. Nevertheless, its wall can be thin enough to permit higher energy betas (>300 keV) to be counted.
The density thickness of a typical thin walled cylindrical GM is 30 mg/cm2. The density thickness (aka aerial density) is a convenient way to describe the thickness of very thin materials—it is the product of the material’s density (mg/cc) and its thickness (cm). To measure it, weigh one square centimeter of the material.
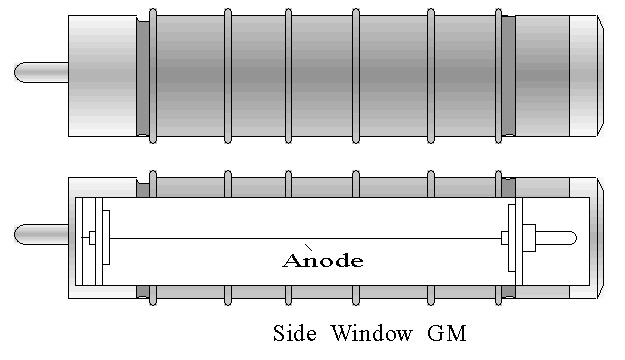
Pancake GM
The pancake GM tube is a truncated cylinder having the shape of a pill box. As is true of the end window GM tube, one end is covered with a thin (usually mica) window. The window thickness is 1.5 to 2.0 mg/cm2.
The shape of the anode is unusual since it has a circular configuration in a plane parallel to the entrance window.
The primary function of the pancake GM is the detection and measurement of beta emitting surface contamination. It also responds to alpha particles and gamma rays.
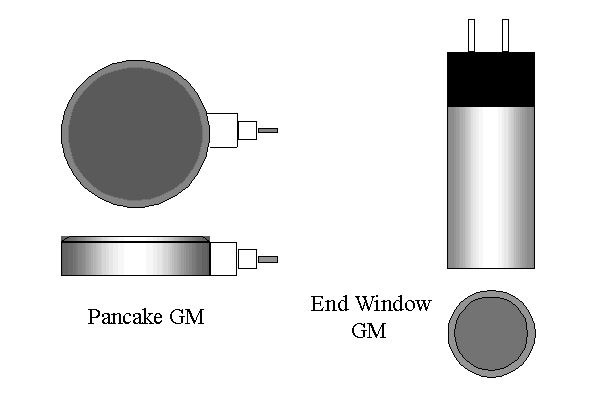
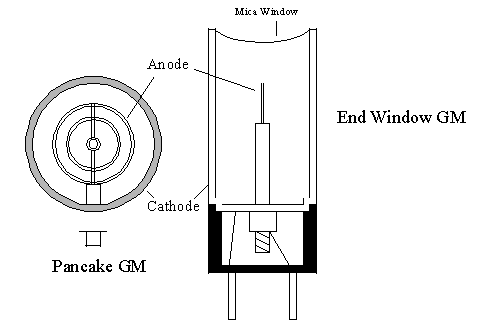
End Window GM
As is the case with the side window GM, the cathode is a stainless steel cylinder. The anode is supported at one end and extends only part way along the tube axis. The tip of the anode is typically covered with a small glass bead. The window, covering one end of the tube, is usually made of mica and typically has a density thickness of 1.5 to 2.0 mg/cm2.
Like any Geiger Mueller detector, the end window GM responds to gamma rays. Nevertheless, the end window GM is most commonly used to count beta activity. The end window GM can also be used to count alpha particles.
Fill Gas
The pressure of the gas inside the GM detector is usually a few tenths of an atmosphere. By reducing the pressure below atmospheric, the strength of the electric field necessary to reach the Geiger-Mueller region, and hence the required operating voltage, is lowered. The reduced pressure also increases the drift velocity of the positive ions towards the cathode and thereby reduces the dead time.
The gas of a Geiger Mueller detector consists of two components: a fill gas and a quench gas.
The fill gas is usually neon but other gases are sometimes used, e.g., helium, argon, or krypton.
There are two main types of quench gas: halogen quench gases and organic quench gases Chlorine is the most common halogen quench gas, but bromine is also used. Although the textbooks usually mention alcohol as an example of an organic quench gas, isobutane is far more common. A halogen quenching agent is used if the fill gas is neon, argon or krypton, while helium filled tubes usually employ an organic quench gas.
Generation of Pulse
If beta particles or alpha particles get through the detector window, they ionize the fill gas directly. Gamma rays and X-rays ionize the gas indirectly by interacting with the metal wall of the GM (via the photoelectric effect, Compton scattering or pair production) in such a way that an electron is "knocked" off the inner wall of the detector. This electron then ionizes the gas inside the tube. It is true that gamma rays or X-rays might interact with the fill gas rather than the wall, but this is less probable except for very low energy photons.
The electric field created by the potential difference between the anode and cathode causes the negative member (electron) of each ion pair to move to the anode while the positively charged gas atom or molecule is drawn to the cathode. As the electrons of the primary ion pairs move towards the anode, they gain kinetic energy (and lose potential energy). If the electric field in the chamber is of sufficient strength (approximately 106 V/m) these electrons gain enough kinetic energy to ionize the gas and create secondary ion pairs. The electrons produced in these secondary ion pairs, along with the primary electrons, continue to gain energy as they move towards the anode, and as they do, they produce more and more ionizations. The result is that each electron from a primary ion pair produces a cascade or avalanche of ion pairs (Townsend avalanche).
At the high voltages associated with the Geiger-Mueller region, the avalanche created by a single primary electron leads to the creation of other "secondary" avalanches, and so many of these "secondary" avalanches occur that they completely envelop the anode. The pulse eventually shuts itself down when the accumulation of positive ions around the anode reduces the strength of the electric field below that required for continued propagation.
The mechanism by which a single avalanche can eventually envelope the entire anode involves the fact that any avalanche not only involves the ionization of gas molecules, it also involves the excitation of gas molecules. The subsequent deexcitation of the excited molecules results in the emission of UV photons. If enough of these photons are produced, some will be absorbed by the gas molecules via the photoelectric effect. This leads to further ionization of the gas and the formation of a second avalanche. For all practical purposes, the multiple avalanches are produced at the same time—only one pulse results. The following diagram shows how a single avalanche can spread along the anode via the emission of UV photons.
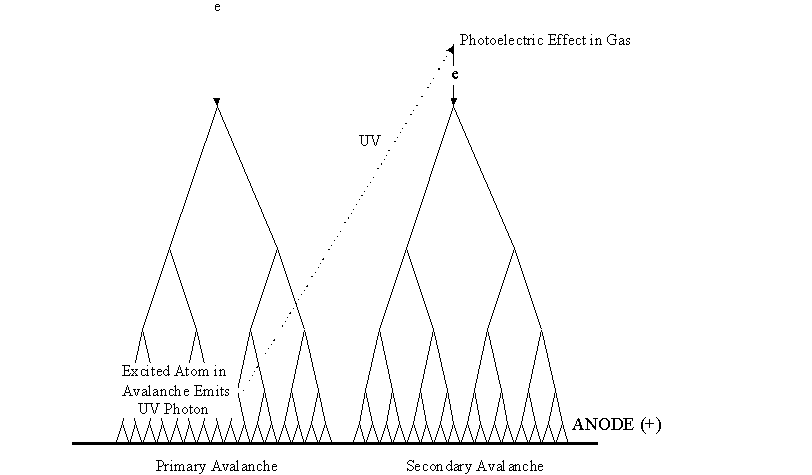
-
20th Century Electronics GM Tube 20th Century Electronics GM Tube
-
Amperex Low-Level Beta Counter Amperex Low-Level Beta Counter
-
Amperex Model 120NB End Window GM Amperex Model 120NB End Window GM
-
Amperex Model 52N GM Tube Amperex Model 52N GM Tube
-
Amperex Model 90NB GM Tube Amperex Model 90NB GM Tube
-
Amperex Models 100NB and 200NB End Window GM Tubes Amperex Models 100NB and 200NB End Window GM Tubes
-
Anton Model 201 End Window GM Tube Anton Model 201 End Window GM Tube
-
Anton Pancake GM With Double Window Anton Pancake GM With Double Window
-
Brain Probe Brain Probe
-
Electronic Products Company Model 5979 End Window GMs Electronic Products Company Model 5979 End Window GMs
-
El-Tronics Model G50S GM Tube El-Tronics Model G50S GM Tube
-
End-Window Geiger Mueller Tubes End-Window Geiger Mueller Tubes
-
Eon Type 7323 Peanut GM Tube Eon Type 7323 Peanut GM Tube
-
GM Detector of Robert Millikan GM Detector of Robert Millikan
-
GM Detectors for Triggering Cloud Chambers GM Detectors for Triggering Cloud Chambers
-
GM Tube of Unknown Manufacture GM Tube of Unknown Manufacture
-
GM Tube of Unknown Manufacture GM Tube of Unknown Manufacture
-
Mystery NCA Tube Mystery NCA Tube
-
Nuclear Chicago D33, D34 and D37A End Window GM Tubes Nuclear Chicago D33, D34 and D37A End Window GM Tubes
-
Pancake/End-Window Hybrid GM Pancake/End-Window Hybrid GM
-
Pancake GM Tubes Pancake GM Tubes
-
Philips GM Tubes Philips GM Tubes
-
Q-Gas Counter Q-Gas Counter
-
Raytheon Model CK 1020 and 1021 GM Tubes Raytheon Model CK 1020 and 1021 GM Tubes
-
RCL End Window GMs, Models 10104, 10105 and 10106 RCL End Window GMs, Models 10104, 10105 and 10106
-
RCL Mark 1 Model 11X GM Tubes RCL Mark 1 Model 11X GM Tubes
-
RCL Mark 1 Model 3 End Window GM RCL Mark 1 Model 3 End Window GM
-
RCL Mark 1 Model 45 GM Tube RCL Mark 1 Model 45 GM Tube
-
RCL Mark 1 Model 5 Probe Counter RCL Mark 1 Model 5 Probe Counter
-
RCL Mark 1 Model 70, Mark 1 Model 71 and the Model 10306 Annular GMs RCL Mark 1 Model 70, Mark 1 Model 71 and the Model 10306 Annular GMs
-
RCL Mark 1 Model 81 Dipping Counter RCL Mark 1 Model 81 Dipping Counter
-
RCL Mark 1 Model 81 Dipping Counter RCL Mark 1 Model 81 Dipping Counter
-
RCL Model 103 (?) GM Tube RCL Model 103 (?) GM Tube
-
RCL Model 10301 GM Tube RCL Model 10301 GM Tube
-
RCL Model 10303 (?) GM Tubes RCL Model 10303 (?) GM Tubes
-
RCL Model 10305 GM Tube RCL Model 10305 GM Tube
-
RCL Model 10399 Peanut GM Tube RCL Model 10399 Peanut GM Tube
-
Technical Associates Models TA-B1 and TA-B3 Thin Wall GMs Technical Associates Models TA-B1 and TA-B3 Thin Wall GMs
-
Tracerlab Glass Wall GM Tracerlab Glass Wall GM
-
Tracerlab Model TGC 315 Annular GM Tracerlab Model TGC 315 Annular GM
-
Tracerlab TGC-1 and TGC-2 End Window GM Tubes Tracerlab TGC-1 and TGC-2 End Window GM Tubes
-
Tracerlab TGC-3A End Window GM Tube Tracerlab TGC-3A End Window GM Tube
-
Tracerlab TGC-5 GM Tube Tracerlab TGC-5 GM Tube
-
Tracerlab TGC-8 GM Tube Tracerlab TGC-8 GM Tube
-
Victoreen Model 1B85 Thyrode Thin Wall GM Victoreen Model 1B85 Thyrode Thin Wall GM
-
Victoreen Model 6306 High Efficiency GM Victoreen Model 6306 High Efficiency GM
-
Victoreen Model VG 10A End Window GM Tubes Victoreen Model VG 10A End Window GM Tubes
-
Victoreen Model VG-18 GM Tube Victoreen Model VG-18 GM Tube